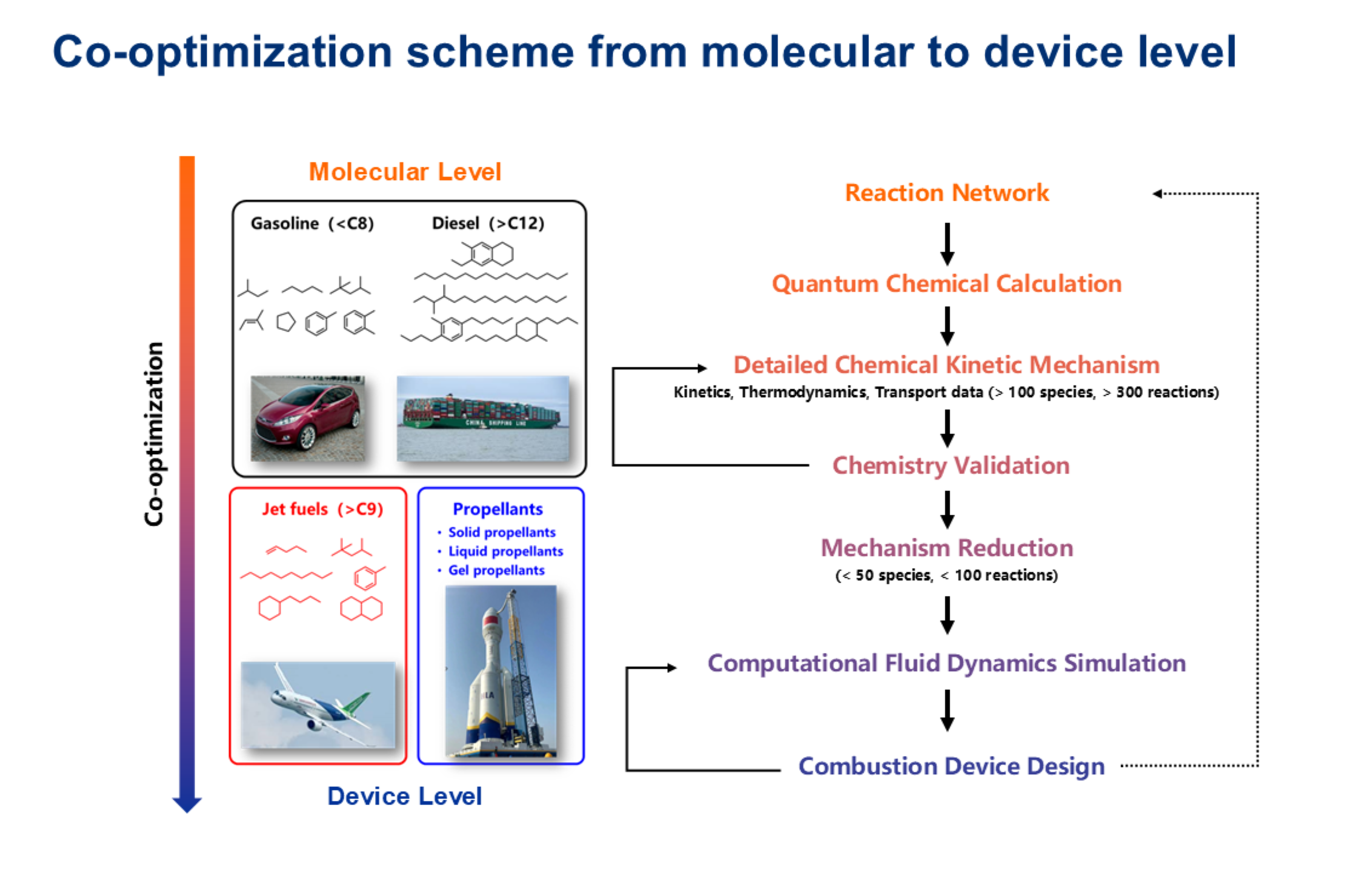
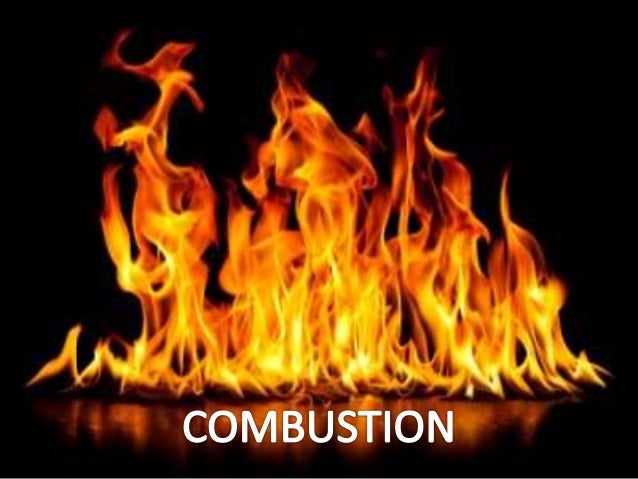
Combustion provides over 80% of the world’s energy and is critical to industrial manufacturing, transportation, and national defence. Understanding the combustion processes of various fuels is critical for designing and optimizing efficient, reliable, and environmentally friendly combustors. Our research adopts a comprehensive approach, spanning molecular to device-level analysis, to achieve co-optimization of fuel properties and combustion performance. At the molecular level, we analyze the fundamental chemical composition and reaction mechanisms of a wide range of fuels, including gasoline, diesel, jet fuels, and solid, liquid, and gel propellants. This involves constructing detailed reaction networks using advanced quantum chemical calculations to derive kinetic, thermodynamic, and transport properties across wide temperature and pressure ranges.
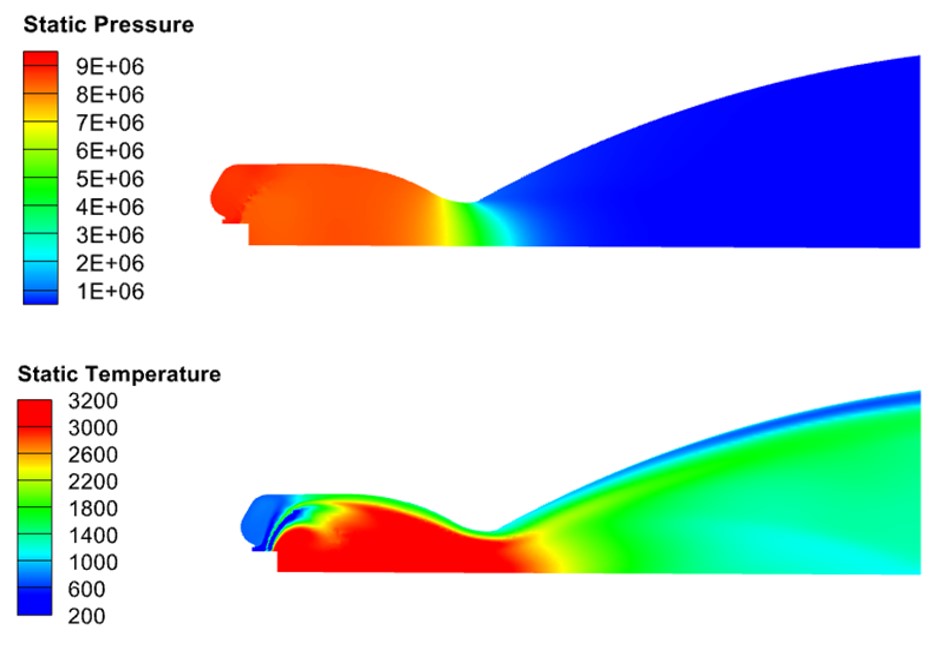
The derived chemical kinetic mechanisms, often involving hundreds of species and reactions, are rigorously validated against experimental data. To ensure computational feasibility for practical applications, these mechanisms are further simplified through reduction techniques, retaining only the most critical species and reactions while maintaining accuracy. The reduced mechanisms are then integrated into computational fluid dynamics (CFD) simulations, enabling the detailed study of Fuel combustion under real-world operating conditions.
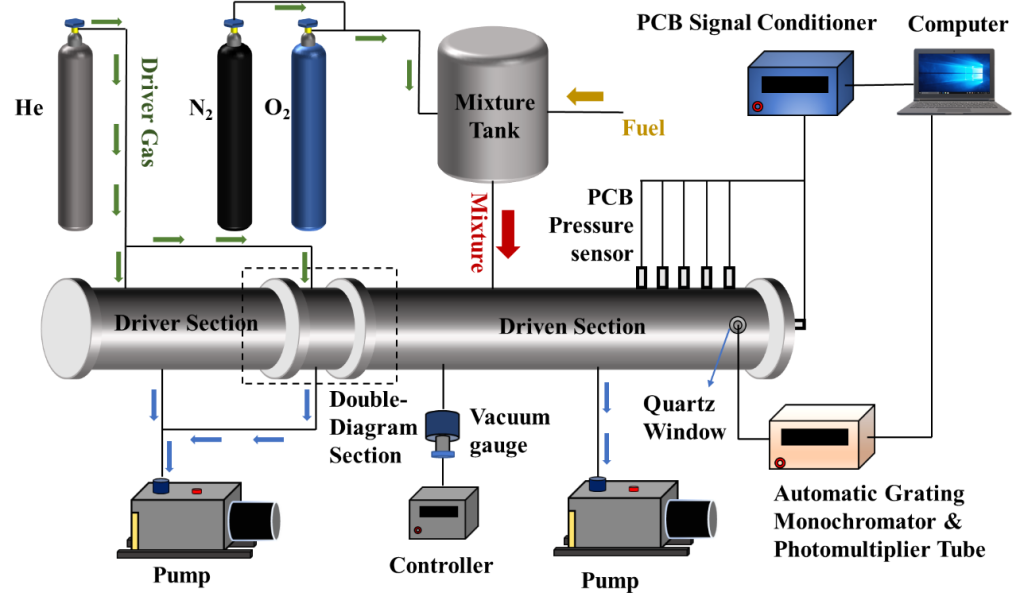
This multi-scale strategy bridges fundamental molecular chemistry with engineering applications, providing a robust foundation for designing and optimizing combustion devices. By iterating between experimental validation, simulation, and mechanism refinement, we aim to enhance the performance, efficiency, and environmental sustainability of combustors, addressing the growing demands for cleaner and more reliable propulsion systems.